One of the biggest remaining mysteries in particle physics is dark matter. It began when scientists observing galaxies found that the observable matter in them (such as stars or clouds of gas) does not produce a large enough gravitational pull to hold itself together; there must be some unseen type of matter present which keeps them together - they called it dark matter. Physicists have proposed several candidates for dark matter and made many attempts to detect them. A new experiment called MIGDAL (Migdal In Galactic Dark mAtter expLoration) at RAL will hopefully help in the search for dark matter by revealing a new channel for direct dark matter detection.
Several dark matters (DM) detectors have been built all around the world, namely a large tank of noble gas cooled down into a liquid state. When a DM particle or neutron enters the tank, it can interact with the atoms in the tank and produce a visible signal with a nuclear recoil. The hope is this signal would come from an unrecognised particle that we could call dark matter. In particular, Migdal events are characterised by two tracks, one belonging to an electron and another to a nuclear recoil, originating from the same vertex. Furthermore, opposite energy loss along both tracks gives a distinctive power to be used against the background events. Adding noble gases will allow the experiment to observe and measure the Migdal effect in the elements relevant to dark matter searches.
The MIGDAL collaboration, which includes members from the PPD DOM group, is aiming to observe this effect and explore its use in DM detection. In particular, the goal is to detect the predicted electron shake-off emission, which is thought to accompany nuclear scattering.
DETECTOR SENSITIVITY
To deal with the first issue of background signals, these detectors have been constructed deep underground using the Earth itself to provide a thick layer of shielding. This blocks out a significant proportion of ordinary particles whereas the potential DM particles, which are much less likely to interact with matter, can reach the detector.
The second issue of detector sensitivity is one with many innovative solutions. One of these is being explored as part of the
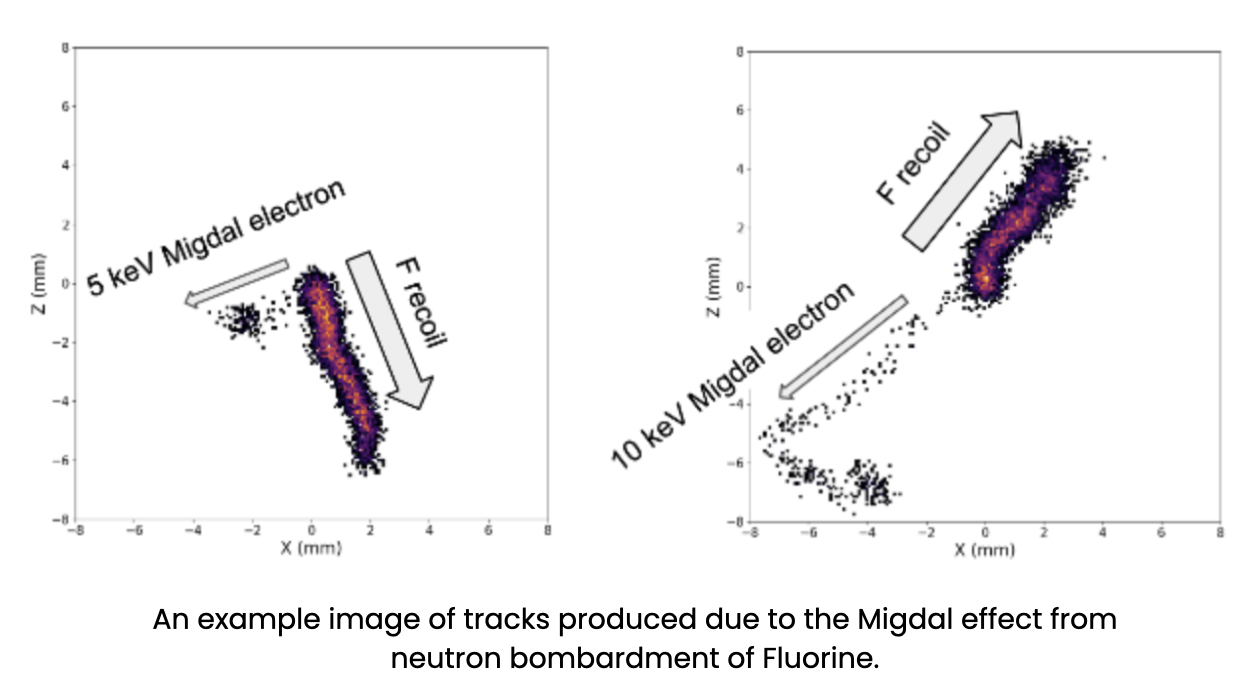
MIGDAL experiment at RAL. To understand it, the principle of a DM detector should first be explained. When a DM particle hits an atom in the detector’s tank it causes the atom’s nucleus to recoil which creates a signal, the strength of which depends on the mass of the DM particle.
There is a limit to how sensitive the detector is, namely, there is a threshold for the signal strength below which it won't be detected. If the DM particle has a low mass (<10 GeV) it will produce a signal below this experimental detection threshold. The Migdal effect would lower this threshold energy: when a nuclear recoil happens because the atom’s surrounding electron cloud will take some time to catch up with the nucleus, there is a small but distinct probability of one of those electrons getting excited and even ionised. This creates the possibility of a DM particle which is below the threshold energy to create a detectable signal but may be able to produce an electron via the Migdal effect. This would widen the range of the energy and mass of DM particles that could be detected.
NILE PROJECT
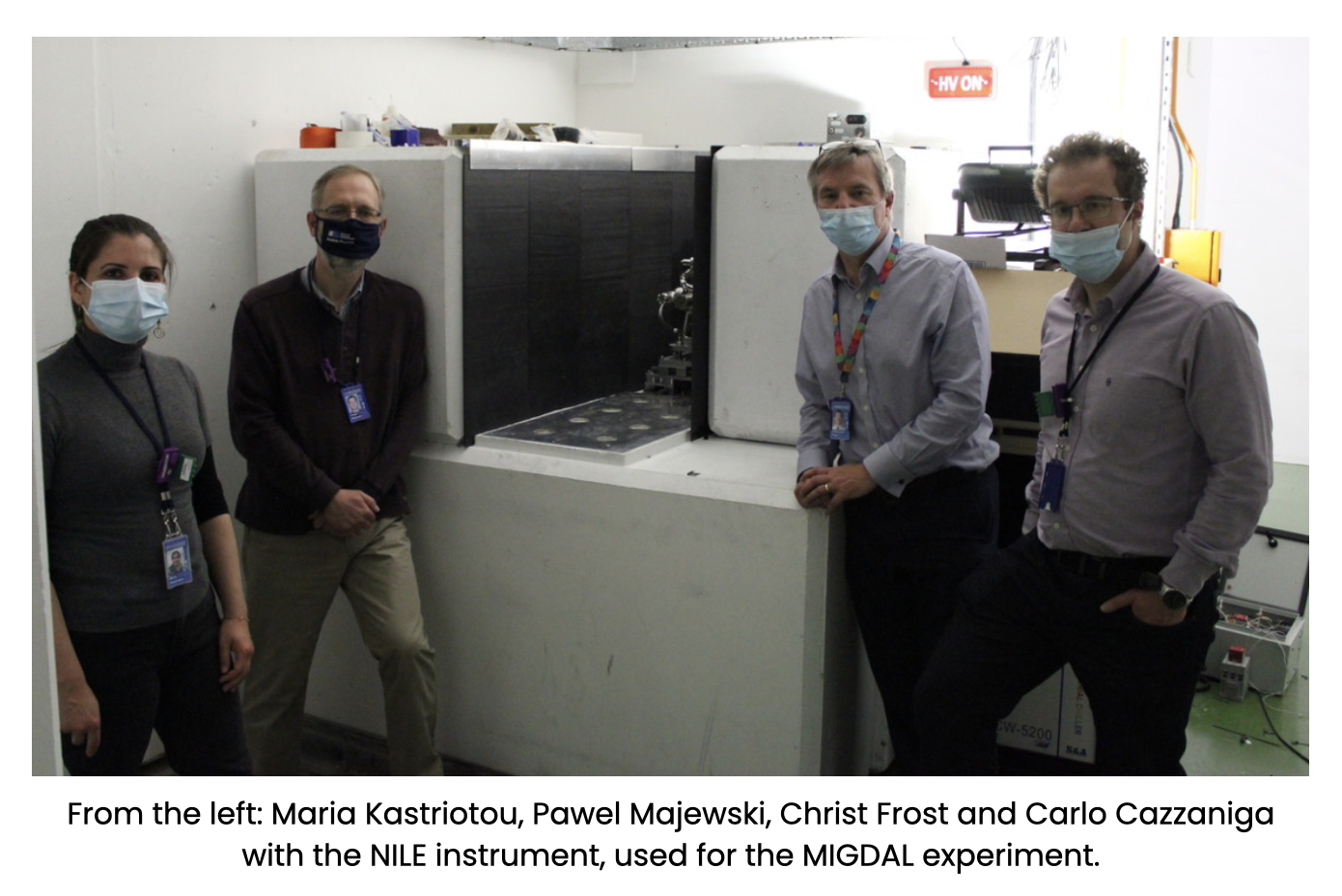
The Neutron Irradiation Laboratory for Electronics (NILE) at ISIS Neutron and Muon Source is a new facility, which had already procured a DT generator (a desktop fusion device using deuterium and tritium to produce high-energy neutrons at 14.1 MeV) to use for testing the resilience of cosmic ray damage test and the effect of space weather on electronics, but they realised the DT generator would be ideally suited for testing the Migdal effect, in which a neutral particle scattering from a nucleus can produce atomic ionisation alongside the nuclear recoil. However, to probe the effect at the low nuclear recoil energies relevant to most dark matter scattering models (up to a few hundred keV) lower energy neutrons are needed from a DD generator (a fusion reactor using only deuterium as a fuel and producing 2.5MeV neutrons), provided in 2021 by UKRI’s World Class Laboratories funding. Following its installation in July 2023, the dark matter detector has accumulated millions of images across the course of 2 science runs. In preparation for its 3rd science run in 2025, the detector has undergone comprehenisve upgrade of various components.